| |
The History of Timekeeping
With respect to human history, timekeeping is a relatively
recent human desire—probably 5000 to 6000 years old. It
was most likely initiated in the Middle East and North Africa.
A clock is defined as a device having two qualities:
- A regular,
constant or repetitive process or action to mark off equal increments of
time. Early examples of such processes included movement of the sun
across the sky, candles marked in increments, oil lamps with marked
reservoirs, sand glasses (hourglasses), and in the Orient, small
stone or metal mazes filled with incense that would burn at a certain pace.
- A means of
keeping track of the increments of time and displaying the result.
Relaying
the history of time measurement has a degree of inaccuracy, much like clocks
themselves. What follows is, if not
completely accurate, as close as many researchers can ascertain.
|
|
Time keeping
has much progressed since its inception,
Time Management
Software now exists as a means to electronically
track and measure time. |
The
Egyptians are the first group of people that we can reasonably prove took
timekeeping seriously as a culture. Many
believe that the Sumerians were thousands of years ahead of the game, but proof
of this is only speculative.
Around 3500 B.C., the Egyptians built obelisks—tall
four-sided tapered monuments—and placed them in strategic locations to cast
shadows from the sun. Their moving
shadows formed a kind of sundial, enabling citizens to partition the day into
two parts by indicating noon. They also showed the year's longest and shortest
days when the shadow at noon was the shortest or longest of the year. Later,
markers added around the base of the monument would indicate further time
subdivisions.
Around
1500 B.C., the Egyptians took the next step forward with a more accurate
shadow clock or sundial. The sundial was divided into 10 parts, with two twilight
hours indicated. This sundial only kept
accurate time (in relative terms) for a half day. So at midday, the device had to be turned 180 degrees to measure
the afternoon hours.
A sundial tracks the apparent movement of the sun around the
earth's celestial pole by casting a shadow (or point of light) onto a surface
that is marked by hour and minute lines. That is why the shadow-casting object
(the gnomon or style) must point towards the north celestial pole, which is very
near Polaris, the North Star. The gnomon serves as an axis about which the sun
appears to rotate.
The sharper the shadow line is, the greater the accuracy. So,
generally speaking, the larger the sundial the greater the accuracy, because the
hour line can be divided into smaller portions of time. But if a sundial gets
too large, a point of diminishing returns is reached because, due to the
diffraction of light waves and the width of the sun's face, the shadow spreads
out and becomes fuzzy, making the dial difficult to read.
In the quest for more year-round
accuracy, sundials evolved from flat horizontal or vertical plates to more
elaborate forms. One version was the hemispherical dial, a bowl-shaped
depression cut into a block of stone, carrying a central vertical gnomon
(pointer) and scribed with sets of hour lines for different seasons. The
hemicycle, thought to have been invented about 300 B.C., removed the useless
half of the hemisphere to give an appearance of a half-bowl cut into the edge of
a squared block.
Back to top
|
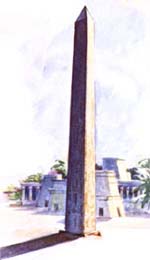
Obelisk
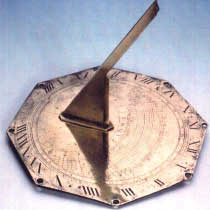
Copper sundial
|
|
ClockWatch time sync software
ClockCard timekeeping PC cards
ClockWatch Star Sync GPS-based time server
ClockWatch Cell Sync CDMA-based time server |
The Egyptians improved upon the sundial with a merkhet, the oldest known astronomical tool.
It was developed around 600 B.C. and uses a string with a weight on the end to accurately measure
a straight vertical line (much like a carpenter uses a plumb bob today). A pair of merkhets were used to establish a North-South line
by lining them up with the Pole Star. This allowed for the measurement of nighttime hours as it measured when certain stars
crossed a marked meridian on the sundial.
By
30 B.C., there were as many as 13 different types of sundials used across
Greece, Asia Minor and Italy.
Back to top
|
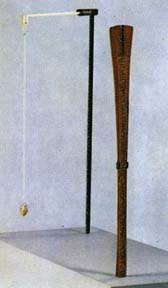
Merkhet |
|
|
Clepsydras or water clocks
were among the first timekeeping devices that didnt use the sun or the
passage of celestial bodies to calculate time. One of the oldest was found in
the tomb of ancient Egyptian King Amenhotep I, buried around 1500 B.C. Around
325 B.C., the Greeks began using clepsydras (Greek for "water thief") by
the regular dripping of water through a narrow opening and accumulating the water
in a reservoir where a float carrying a pointer rose and marked the hours. A
slightly different water clock released water at a regulated rate into a bowl until it
sank. These clocks were common across the Middle East, and were still being used in
parts of Africa during the early 20th century. They could not be relied on to
tell time more closely than a fairly large fraction of an hour.
More elaborate and impressive mechanized water clocks were developed between 100 B.C.
and 500 A.D. by Greek and Roman horologists and astronomers. The added
complexity was aimed at making the flow more constant by regulating the
pressure providing fancier displays of the passage of time. Some water
clocks rang bells and gongs; others opened doors and windows to show little
figures of people, or moved pointers, dials, and astrological models of the
universe. More on Roman timekeeping...
A Greek astronomer, Andronikos, supervised the construction of
the Tower of the Winds in Athens in the firstst century B.C. This octagonal
structure showed scholars and marketplace shoppers both sundials and mechanical
hour indicators. It featured a 24-hour mechanized clepsydra and indicators for
the eight winds from which the tower got its name, and it displayed the seasons
of the year and astrological dates and periods.
In the Far East, mechanized
astronomical/astrological clock-making developed from 200 to 1300 A.D.
Third-century Chinese clepsydras drove various mechanisms that illustrated
astronomical phenomena. One of the most elaborate clock towers was built by Su Sung
and his associates in 1088 A.D. Su Sung's mechanism incorporated a
water-driven escapement invented about 725 A.D.
The Su Sung clock tower, over 30 feet tall,
possessed a bronze power-driven armillary sphere for observations, an
automatically rotating celestial globe, and five front panels with doors that
permitted the viewing of mannequins which rang bells or gongs, and held tablets
indicating the hour or other special times of the day.
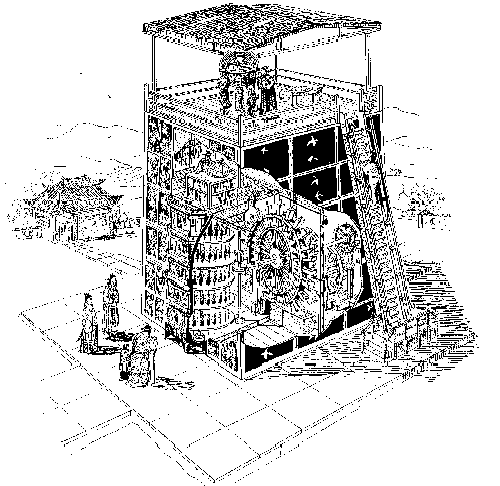
Su Sung's clock tower, ca. 1088
Back to top
|
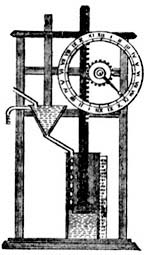
Water clock
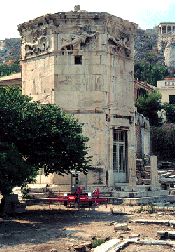
Tower of the Winds, Athens, Greece
|
|
ClockWatch time sync software
ClockCard timekeeping PC cards
ClockWatch Star Sync GPS-based time server
ClockWatch Cell Sync CDMA-based time server
|
The mechanical clock was probably
invented in medieval Europe. Clever
arrangements of gears and wheels were devised that turned by
weights attached to them. As the weights were pulled downward by the force of
gravity, the wheels were forced to turn in a slow, regular manner. A pointer,
properly attached to the wheels, marked the hours.
These clocks became common in
churches and monasteries and could be relied upon to tell when to toll the bells
for regular prayers or church attendance. (The very word "clock" is
from the French cloche, meaning "bell.")
Eventually, mechanical clocks were
designed to strike the hour and even to chime the quarter-hour. However, they
had only an hour hand and were not enclosed. Even the best such clocks would
gain or lose up to half an hour a day.
A technological advance came with
the invention of the spring-powered clock around 1500-1510, credited to
Peter Henlein of Nuremberg, Germany. Because
these clocks could fit on a mantle or shelf they became very popular among the
rich. They did have some time-keeping
problems, though, as the clock slowed down as the mainspring unwound. The
development of the spring-powered clock was the precursor to accurate timekeeping.
In 1582, Italian scientist Galileo,
then a teenager, had noticed the swaying chandeliers in a cathedral. It seemed
to him that the movement back and forth was always the same whether the swing
was a large one or a small one. He timed the swaying with his pulse and then
began experimented with swinging weights. He found that the "pendulum"
was a way of marking off small intervals of time accurately.
Once Galileo had made the discovery,
the regular beat of the pendulum became the most accurate source used to
regulate the movement of the wheels and gears of a clock.
It wasn't a perfect system, though,
as a pendulum swings through the arc of a circle, and when that is so, the time
of the swing varies slightly with its size. To make the pendulum keep truly
accurate time, it must be made to swing through a curve known as a
"cycloid."
In 1656, Dutch astronomer Christian
Huygens first devised a successful pendulum clock.
He used short pendulums that beat several times a second, encased the
works in wood, and hung the clock on the wall. It
had an error of less than one minute a day. This was a huge improvement on earlier mechanical clocks, and
subsequent refinements reduced the margin of error to less than 10 seconds per
day.
In 1670, English clockmaker William
Clement made use of a pendulum about a yard long that took a full second to move
back and forth, allowing greater accuracy than ever before. He encased the
pendulum and weights in wood in order to diminish the effect of air currents,
thus was born the "grandfather's clock." For the first time, it made
sense to add a minute hand to the dial, since it was now possible to measure
time to the nearest second.
In 1721, George Graham improved the
pendulum clocks accuracy to within a second a day by compensating for changes
in the pendulum's length caused by temperature variations. The mechanical clock
continued to develop until it achieved an accuracy of a hundredth of a second a
day and it became the accepted standard in most astronomical observatories.
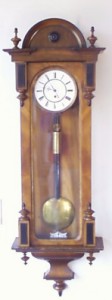
Wall Clock from the 1870s
Back to top
|
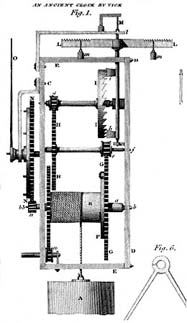
Early mechanical clock
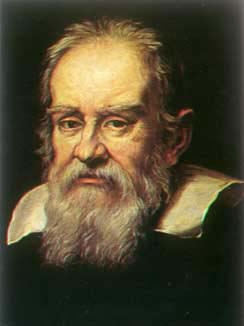
Galileo
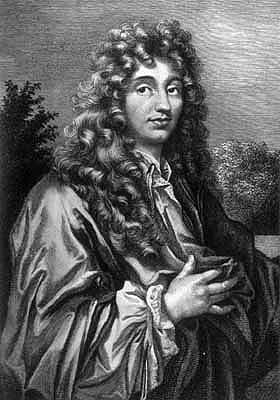
Christian Huygens
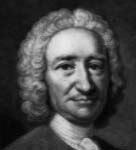
George Graham
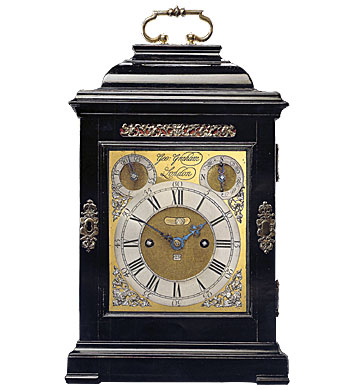
Early Graham clock
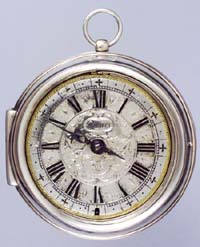
17th Century pocket watch
|
Quartz
Clocks
|
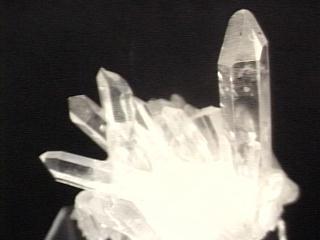
a quartz crystal
chemical name: SiO2, Silicon dioxide
|
|
ClockWatch time sync software
ClockCard timekeeping PC cards
ClockWatch Star Sync GPS-based time server
ClockWatch Cell Sync CDMA-based time server
|
The
running of a quartz clock is based on an electric property of the quartz
crystal. When an electric field is applied to a quartz crystal, it changes the
shape of the crystal itself. If you then squeeze it or bend it, an electric
field is generated. When placed in an electronic circuit, the interaction
between the mechanical stress and the electrical field causes the crystal to
vibrate, generating a constant electric signal which can then be used to measure
time.
Quartz clocks continue to dominate
the market because of the accuracy and reliability of their performance and by
their low cost when produced in mass quantities.
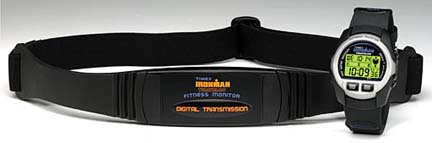
A modern quartz digital watch that not only
keeps accurate time,
but can check your heart rate, too.
The Black Watch,
released in 1975 by the Sinclair Company, was one of the first digital watches
ever produced, and probably the worst. If you were unlucky enough to
buy one of these lemons you could expect various kinds of trouble: |
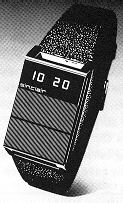 |
- The internal chip could be ruined
by static from your nylon shirt, nylon carpets or air-conditioned
office. This problem also affected the production facility, leading to
a large number of failures before the watches even left the factory.
The result was that the display would freeze on one very bright digit,
causing the batteries to overload (and occasionally explode).
- The accuracy of the
quartz timing crystal was highly temperature-sensitive; the watch ran
at different speeds in winter and summer.
- The batteries had a life
of just ten days; this meant that customers often received a Black
Watch with dead batteries inside. The design of the circuitry and case
made them very difficult to replace.
- The control panels
frequently malfunctioned, making it impossible to turn the display on
or off, which again led to
exploding batteries.
- The watch came in a kit
which was almost impossible for hobbyists to construct. Practical
Wireless magazine advised readers to use two wooden clothes pegs,
two drawing pins and a piece of insulated wire to work the batteries
into position. You then had to spend another four days adjusting the
trimmer to ensure that the watch was running at the right speed.
- The casing was
impossible to keep in one piece. It was made from a plastic which
turned out to be unglueable, so the parts were designed to clip
together—which they didn't.
- A very high percentage
of Black Watches were returned, leading to the legend that Sinclair
actually had more returned than had been manufactured. The backlog
eventually reached such monstrous proportions that it still hadn't
been cleared two years later.
Back to top
|
|
|
|
ClockWatch time sync software
ClockCard timekeeping PC cards
ClockWatch Star Sync GPS-based time server
ClockWatch Cell Sync CDMA-based time server |
Termed NIST F-1, the cesium
atomic clock at the National Institute of Science and Technology (NIST), in
Boulder, Colorado is the nation's primary frequency standard that is used to
define Coordinated Universal Time (known as UTC), the official world time.
Because NIST F-1 shares the distinction of being the most accurate clock in the
world (with a similar device in Paris), it is making UTC more accurate than ever
before. NIST F-1 recently passed the evaluation tests that demonstrated it is
approximately three times more accurate than the atomic clock it replaces,
NIST-7, also located at the Boulder facility. NIST-7 had been the primary atomic
time standard for the United States since 1993 and was among the best time
standards in the world.
NIST F-1 is referred to as a
fountain clock because it uses a fountain-like movement of atoms to obtain its
improved reckoning of time. First, a gas of cesium atoms is introduced into the
clock's vacuum chamber. Six infrared laser beams then are directed at right
angles to each other at the center of the chamber. The lasers gently push the
cesium atoms together into a ball. In the process of creating this ball, the
lasers slow down the movement of the atoms and cool them to near absolute zero.
Two vertical lasers are used to
gently toss the ball upward (the "fountain" action), and then all of
the lasers are turned off. This little push is just enough to loft the ball
about a meter high through a microwave-filled cavity. Under the influence of
gravity, the ball then falls back down through the cavity.
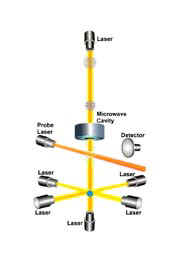
The fountain action of the cesium clock
As the atoms interact with the
microwave signaldepending on the frequency of that signaltheir atomic
states may or may not be altered. The entire round trip for the ball of atoms
takes about a second. At the finish point, another laser is directed at the
cesium atoms. Only those whose atomic states are altered by the microwave cavity
are induced to emit light (known as fluorescence). The photons (tiny packets of
light) emitted in fluorescence are measured by a detector.
This procedure is repeated many
times while the microwave energy in the cavity is tuned to different
frequencies. Eventually, a microwave frequency is achieved that alters the
states of most of the cesium atoms and maximizes their fluorescence. This
frequency is the natural resonance frequency for the cesium atomthe
characteristic that defines the second and, in turn, makes ultra precise
timekeeping possible.
The "Natural frequency" recognized
currently as the measurement of time used by all scientists, defines the period
of one second as exactly 9,192,631,770 oscillations or 9,192,631,770 cycles of
the Cesium Atom's Resonant Frequency. The cesium-clock at NIST is so accurate
that it will neither gain nor lose a second
in 20 million years!
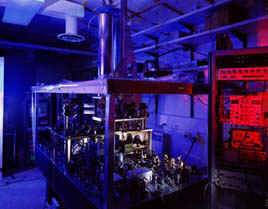
The cesium atomic clock at the NIST
This new standard is more accurate
by a wide margin than any other clock in the United States and assures the
nation's industry, science and business sectors continued access to the
extremely accurate timekeeping necessary for modern technology-based operations.
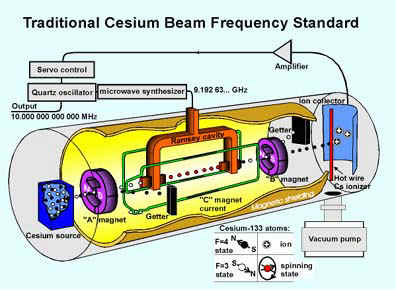
Back to top
|
|
ce·si·um
(s z - m).
n. r>
Symbol Cs
A soft, silvery-white ductile metal, liquid at room temperature, the
most electropositive and alkaline of the elements, used in photoelectric
cells and to catalyze hydrogenation of some organic compounds. Atomic
number 55; atomic weight 132.905; melting point 28.5°C; boiling point 690°C;
specific gravity 1.87; valence 1.
|
|
Cesium was discovered by spectroscopy in
1860 by Robert Bunsen and Gustav Kirchhoff.
One gram of cesium is an ample supply for a typical atomic clock to run
for one year.
A gram of cesium could be found in about a cubic foot of ordinary granite. Natural cesium is pure cesium-133 (55 protons and 78 neutrons in the
nucleus, 55+78=133): it is non-radioactive. |
ClockWatch time
sync software
ClockCard timekeeping PC cards
ClockWatch Star Sync GPS-based time server
ClockWatch Cell Sync CDMA-based time server |
Links to Clock, Watch, Time and
Horology Websites |
ClockWatch –
Atomic clock-based time sync software
Contemporary and stylish
sterling silver clocks
at Silvergroves.co.uk
Star Sync – get precise time from
GPS satellites
Cell Sync – time from CDMA cellular transmitters
Radio Sync – WWVB time signal radio receiver
ClockCard – timekeeping PC cards
ExactSet – radio controlled clocks
|
|
Back to top
Back
to main page |
|
| | |